Winter Lightning in the Southeast U.S. and its Relation to Heavy Frozen Precipitation
by
Steven M. Hunter, S. Jeffrey Underwood, Ronald L. Holle, and Thomas L. Mote
1. Introduction
- Winter or cool season storms across the southeastern United States are not as common as in other areas of the country. This modest frequency, however, contributes to the potential disruption of human activity in the region from frozen precipitation (Rooney 1967). Heavy snowfalls in the Southeast have been analyzed and synoptic conditions favorable for their development have been identified (Mote et. al. 1997). Further, the synoptic and dynamic processes favorable for heavy snowfall have been examined for other regions of the country (Uccellini and Kocin 1987; Kocin and Uccellini 1990; Kocin et. al. 1995). There are, however, limitations to forecasting/nowcasting complex winter precipitation and convection across the southeast and mid-Atlantic U.S. This complexity is the result, in part, of the topography of the region and the prevalence of Appalachian Cold Air Damming (CAD) during the winter months (Keeter and Cline 1991).
- The potential of cloud-to-ground (CG) lightning detection networks to augment existing radar, radiosonde, and satellite technologies in forecasting and nowcasting frozen precipitation has been the subject of almost no research. Such augmentation seems viable, however, since the Southeast has adequate CG lightning detection network coverage and a higher incidence of convection during the cool season. At the very least, near-real-time lightning flash data should be useful for detecting the initiation, existence or trend of winter season convection, identifying embedded convection, and diagnosing conditional symmetric instability (CSI).
- Climatological diagnoses of winter lightning have identified flash density maxima over the Gulf Stream off the east coast (Orville 1990) and the central U.S. (Holle et al., 1998). Most of the few studies of lightning in winter, however, have focused on single storms. Holle et al. (1997) analyzed a single isolated flash that destroyed a Connecticut home in February. Orville (1993) examined the "Blizzard of '93," which produced prodigious CG lightning in its warm sector. Roohr and Vonder Haar (1996) found a correlation between lightning and heavy snow in a March storm in Colorado. Studwell and Orville (1995) studied lightning patterns in a severe ice and snow storm over the Southeast and Atlantic coast. They discovered a predominance of positive flashes (delivering net positive charge to ground) in areas of freezing rain. A similar association was made by Holle and Watson (1996) for two central U.S. events.
- This research will address some of the shortcomings in the current knowledge of winter lightning, by documenting the primary atmospheric processes that accompany such lightning across the southeast U.S. We will investigate relationships of lightning flash density, polarity and multiplicity with location, intensity, duration and phase of winter precipitation. We will also focus on processes resulting in cold season convection and Conditional Symmetric Instability (CSI), as well as those that distinguish cases with abundant lightning vs those with little lightning. Finally, we will diagnose the meteorological environment, particularly as revealed by vertical stability and motions, in the hope that some predictive tools for major winter storms may result.
2. Data and Methodology
- The initial approach will use case studies. Seven cases have been identified from a population of winter precipitation events across the southern and eastern U.S. The seven events are catalogued in Table 1. The study area is defined by an area between 25N latitude and 40N latitude, and 75W longitude and 95W longitude. The study area is shown in Fig. 1. All cases had major impacts, owing to heavy accumulation of ice or snow in the area.
Event #, Date |
Affected States |
Storm Description |
1. Feb. 9-11, 1994 |
MS, AR, TN, AL, TX |
$300M ice storm |
2. January 6-7, 1995 |
AR, TN, AL, MO, KY, IL |
Freezing rain, ice pellets |
3. Feb. 3-4, 1995 |
VA, NC, GA |
Mainly heavy snow; little lightning |
4. January 1-2, 1996 |
AR, MO, TX |
Mainly heavy snow |
5. Feb. 1-3, 1996 |
TX, OK, LA, AR, MS, TN, AL, KY, GA, NC, OH |
Widespread heavy ice & snow |
6. Jan. 9-10, 1997 |
SC, NC, VA, MD, WV, TN |
Freezing rain, ice pellets; cold air damming |
7. January 27, 1997 |
MO, IL, IN, KY, OH |
Heavy snow & ice |
Table 1. Dates and precipitation descriptions of numbered case studies.
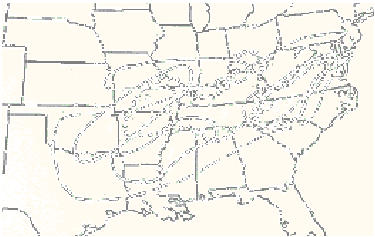
Figure 1. Study area and spatial extent of the areas most severely impacted with frozen precipitation.
3. Preliminary Results
- Although one cannot claim climatological representativeness from seven cases, the geographic locations and orientations of the impact areas in Fig. 1 are suggestive, since there is a large area of overlap between them. Moreover, all but one have a southwest-northeast orientation. The overlap region intimates that it may be favored for baroclinic zones and isentropic or other types of lift. A review of the synoptic meteorology of the cases, however, reveals that there is significant diversity between them.
- Table 2 briefly summarizes major findings of the synoptic analyses, specifically the primary mechanisms for vertical motion and characterizations of the cold air masses involved.
Case Number (after Table 1) |
Dominant Lifting Mechanisms, Synoptic Features & Cold Air Information |
1 |
Isentropic lift over shallow arctic airmass, plus upper divergence associated with jet, possibly CSI/frontogenesis |
2 |
General isentropic lift, strong warm air advection (WAA) with low-level jet (LLJ) ahead of developing cyclone |
3 |
Upslope flow, advecting and lifting "wraparound" moisture in cold sector of cyclone |
4 * |
Cyclone dynamics with local enhancement by upslope flow; just enough cold air for snow in NW quadrant of cyclone |
5 * |
Upper divergence associated with jet, plus isentropic lift over arctic airmass into Gulf Coast states |
6 |
Isentropic lift, strong WAA over dammed cold air in mid-Atlantic states. LLJ collocated with maximum WAA |
7 * |
Isentropic lift, strong WAA over strong east-west arctic front |
Table 2. Case study numbers and characterization of predominant lifting mechanisms (most important mentioned first) and cold air involvement. Asterisks near case numbers signify that a preliminary analysis of lightning and radar data has been completed.
- The table reveals that in five of seven cases, isentropic lift was either a primary or secondary cause of upward vertical motion and resulting precipitation. In three of these five cases, the isentropic upglide occurred over an arctic airmass, which was deeper over the central Plains than areas further east. The isentropic motion is directly associated with low-level warm air advection (Doswell, 1982). As evinced by Table 1, the five isentropic cases involved a mixture of frozen precipitation, i.e. ice and snow. This mixture indicates dependence on the depth of cold air at a particular location.
- In contrast, the "non-isentropic lift" cases (3 and 4) exhibited heavy frozen precipitation to the rear of cyclones, in their cold sectors. Surprisingly, deeper exploration showed that upslope flow was a major contributor to precipitation amounts, at least over localized regions. In case 3 this flow was forced by the Appalachian chain, and in case 4 by the Ozarks. Furthermore, the precipitation in both cases fell almost exclusively in the form of snow (Table 1).
- For cases 4, 5 and 7, we have performed preliminary inspections of lightning and radar data. While too early to make far-reaching conclusions, some patterns were found.
- In the isentropic/arctic cases 5 and 7, the great majority of ground flashes were associated with southwest-to-northeast convective lines with maximum reflectivities 40-50 dBZ. These lines were aligned parallel to the mean wind in the cloud-bearing layer, and were usually found on the warm side of the surface arctic front. They were, however, very close to the surface freezing line (0C). To the north, very close to or just north of the front, was a "stratiform" precipitation region that typically had lower peak reflectivities, 30-40 dBZ. The convective echoes often advected into and merged with the southern flank of the stratiform echo, where flashes were much fewer in number. In case 7, and possibly case 5, there was ~10 dB enhancement in echo intensity in the stratiform area to the northeast (downwind) of convective lines. There were some differences in the two cases. In case 7 the arctic boundary was west-east. In case 5 the boundary was oriented southwest-northeast and was located much further south. This may help explain why it generated far more lightning than case 7, as the warm sector was over the moist, unstable waters of the Gulf of America.
- Case 4 was a study in contrast, involving a northeast-moving cyclone that produced a swath of heavy snow in its northwest sector. Lightning was numerous and concentrated in clusters just east of the surface low, commonly associated with 50-60 dBZ echoes. The clusters were in the warm sector, some 500 km from the surface freezing isotherm. Therefore there was no frozen precipitation anywhere near the ground flashes. Also, isentropic lift and warm air advection in the lightning area were minimal, as there was weak low-level flow and no strong thermal boundary. There were no flashes in the stratiform area (~20-30 dBZ) producing the snow swath.
- Flash frequency and polarity was studied in case 7 for those flashes within range (230 km) of the three WSR-88D radars in MO. For the time of heaviest precipitation (0900-1400 UTC), flash rates were 22 h-1 in western MO and 40 h-1 in the eastern part of the state. This rate difference had some correlation to precipitation amounts, as Columbia and St. Louis in the east had over three times as much precipitation as Kansas City in the west. The ratio of positive to total flashes was 30-40%. This is much higher than the average winter percentage of about 5% (Orville and Silver, 1997). No horizontal segregation of positive and negative flashes, such as a bipole pattern (Orville et al., 1988) was apparent. We hope to have the frequency and polarity figures for cases 4 and 5 shortly.
4. Discussion and Future Work
- Despite the early stage of our work, some suggestions are emerging from the data. Although preliminary lightning and radar analyses have only been done for three cases, it may be that connections between lightning and precipitation may only be made for the isentropic lift type cases. In such cases, electrically active convective areas upstream may augment frozen precipitation in the stratiform precipitation area in and above a cold (often arctic) air mass. Indeed, in some instances elevated convection may occur above such air masses if there is sufficient buoyancy with the mechanically lifted air.
- Whatever their location relative to the cold air, the convective cloud masses may "seed" the downwind stratiform cloudiness with large ice crystals. These particles could then grow by accretion/aggregation and subsequently melt/refreeze before reaching the surface, or upon impact. The frequency of ground flashes likely signals the intensity of the convection and may serve as a predictor of the downwind frozen precipitation enhancement. We have not done enough study to say if polarity information such as positive ratios will provide any predictive capability for heavy freezing precipitation. To answer these and other questions, further research is planned.
- We will further refine our description of synoptic and mesoscale circulations, and determine the depth of cold air in the impacted areas. Stability profiles of lifted air will be examined to determine the presence and nature (e.g., upright vs slantwise) of convection. The depth of the lifted warm air (> 0C) will be compared between cases using sounding data, to ascertain any correspondence to precipitation amounts. Correlation of flash patterns to radar echo intensities and organization will continue, to determine added value of lightning data over radar data in these situations. Flash frequency (h-1) will be compared for all cases to see if there are differences in the meteorological processes between prodigious flash-producing storms and those with modest flash densities. We will stratify the positive flash ratios by surface temperature to see if they increase with decreasing temperature (as ratios do between summer and winter).
5. Acknowledgments
- We are deeply indebted for data and software support to Mace Bentley of the University of Georgia Climatology Research Lab, and Kurt Hondl and Karen Cooper of the National Severe Storms Laboratory.
6. References
- Doswell, C.A., 1982: The operational meteorology of convective weather, Vol. 1. NOAA Technical Memorandum NWS NSSFC-5. Available from the National Technical Information Service.
- Holle, R.L., and Watson, A.I., 1996: Lightning during two central U.S. winter precipitation events. Wea. and Forecasting, 11, 4, 599-614.
- ____, J.V. Cortinas Jr., and C.C. Robbins, 1998: Thunderstorms in the United States. Preprints, 19th Conf. Severe Local Storms, Minneapolis, Amer. Meteor. Soc, 298-300.
- Keeter, K.K., and J.W. Cline, 1991: The objective use of observed and forecast thickness values to predict precipitation type in North Carolina. Wea. and Forecasting, 6, 456-469.
- Kocin, P.J., and L.W. Uccellini, 1990: Snowstorms along the Northeastern coast of the United States; 1955 to 1985. Amer. Meteor. Soc., 280 pp.
- ____, P.N. Schumacher, R.F. Morales Jr., and L.W. Ucellini, 1995: Overview of the 12-14 March 1993 superstorm. Bull. Amer. Meteor. Soc., 76, 165-182.
- Mote, T.L., D.W. Gamble, S.J. Underwood, and M.L. Bentley, 1997: Synoptic-scale features common to heavy snowstorms in the southeast United States. Wea. and Forecasting, 12, 1, 5-23.
- Orville, R.E., R.W. Henderson, and L.F. Bosart, 1988: Bipole patterns revealed by lightning locations in mesoscale storm systems. Geophys. Res. Letters, 15, 129-132.
- ____, 1990: Winter lightning along the East Coast. Geophys. Res. Letters, 17, 713-715.
- ____, 1993: Cloud-to-ground lightning in the blizzard of '93. Geophys. Res. Letters, 20, 1367-1370.
- ____, and A.C. Silver, 1997: Lightning ground flash density in the contiguous United States: 1992-95. Mon. Wea. Rev., 125, 631-638.
- Roohr, P.B., and T.H. Vonder Haar, 1996: Lightning in winter storm convection: Initial findings and examination of 5 Mar 1990 Colorado "storm of the century." Preprints, 15th Conf. on Wea. Analysis and Forecasting, Norfolk VA, Amer. Meteor. Soc., 64-67.
- Rooney, J.F., 1967: The urban snow hazard in the United States: An appraisal of disruption. Geographic Rev., 57, 538-559.
- Studwell, A.M., and R.E. Orville, 1995: Characteristics of cloud-to-ground lightning in a severe winter storm, 9-12 February 1994. Preprints, 6th Conf. Aviation Wea. Systems, Dallas, Amer. Meteor. Soc, 176-181.
- Uccellini, L.W., and P.J. Kocin, 1987: Interaction of jet streak circulation during heavy snow events along the east coast of the United States. Wea. and Forecasting, 2, 289-308.