Type 2: External Boundary/Convergence Line Intersecting the
Central or South-central End of a Convective Line. (Intensifying Stage)
|
(1) 4 of 15 cases studied supported this type of reflectivity
pattern.
- External boundary(ies) are identified by:
a) reflectivity fine line or
b) small isolated cells oriented orthogonal (usually east-west or southeast-
northwest) intersecting the central or south-central part of the
convective line.
- 4 warm season events
3 late night/early morning.
1 late afternoon.
|
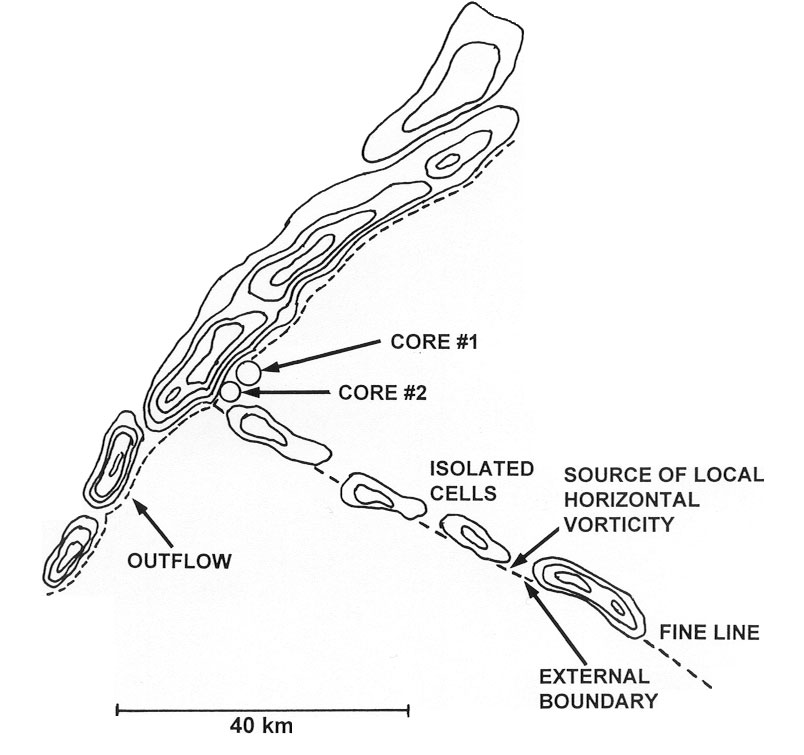
Conceptual Model of Type II Severe Wind MCSs.
|
(b) In the four cases studied, initial bowing of the convective line and first reports of damaging
winds occurred just south of the intersection of the external boundary and linear convective line.
- In each of the four cases, damaging winds were not reported north of the intersection.
-The MARC velocity signature was found in each of the four cases, mainly identified south
of the intersection during this stage of MCS evolution.
-Boundaries we speak of appear not to be associated with relatively large areas of precipitation
(where the air north of the boundary may not be saturated). Rather temperature gradients of 5K or
greater across a boundary may be present. (Markowski et al 1998)
|
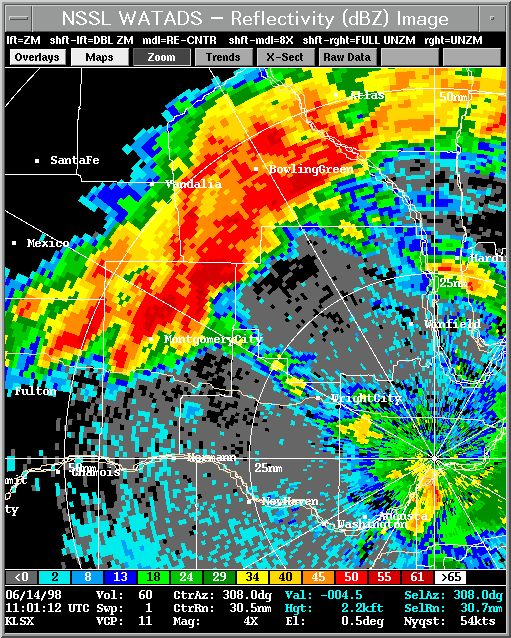
Above - Plan view of 0.5 degree slice WSR-88D
reflectivity image at 1101 UTC from KLSX.
|
Example of the Type 2 pattern is shown left on the reflectivity image 1101 UTC, 14 June 1998 MCS case across east-central Missouri.
- The boundary is identified by small isolated convective cells oriented orthogonal (southeast-northwest)
intersecting the southern part of the convective line.
- Passage of the external boundary over KLSX caused a significant change in the local low-level wind shear profile. Magnitudes of 0-2 (0-3) km storm-relative helicity
increased from 340 (500) m2/s2 at 1025 UTC to 670 (993) m2/s2 at 1111 UTC. Rasmussen (personal
communications) stated that old external boundaries may lose their thermal properties with time, however helical flow will persist for an extended period of time.
- Note the intensifying convective cell just north of the intersection.
|
(c) Characteristics of convective-scale vortices (Type 2 systems)
- In 2 of the 4 cases documented, 1st and 2nd core vortices formed just after an isolated cell, anchored along an
external boundary, merged with the convective line. In both cases, non-supercell tornadoes occurred after the merger.
|
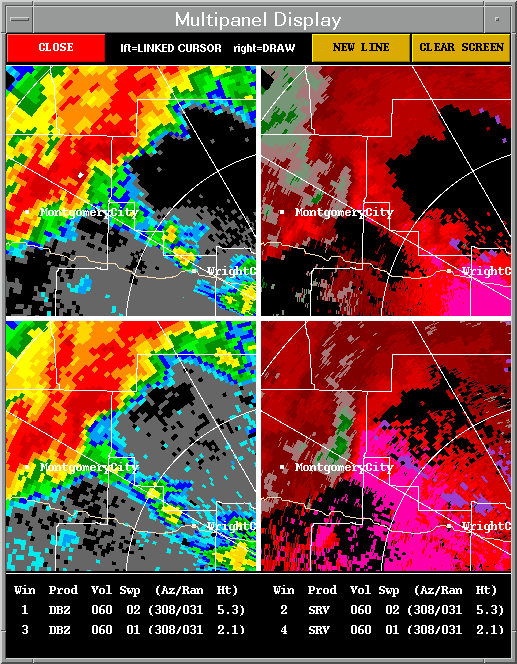
Above - Four panel reflectivity/storm relative velocity
presentation from KLSX at 1101 UTC. Upper two slices 1.5 degrees/lower two slices 0.5 degree slice.
|
Circulation Trends:
- First convective-scale vortex (core #1)formed just north of the intersection between the convective line and (isolated cell) external boundary.
This circulation may exhibit either a:
1) nearly a persistent depth or
2) non-descending characteristics.
- Second convective-scale vortex (core #2) will frequently form just south of the first vortex. This circulation becomes the strongest and most persistent of the group of vortices identified with the MCS.
- Early stages of this circulation will reveal 'non-descending' characteristics. This vortex will often rapidly intensify and deepen within the first 20 minutes of the
circulation's lifespan. Yet the strongest cyclonic shears will often be detected within the lowest 2 km of the circulation's depth.
|
(d) Non-supercell tornadogenesis:
- In three of the four cases studied, weak tornadoes (F0 / F1 intensity) occurred in the vicinity of the 2nd core and within 15 km on
the 'cool side' of the external boundary. Isolated cell-convective line mergers occurred in 2 of these 4 cases.
- Tornadoes occurred during the later part of the 'Organizing Stage' and/or very early part of the 'Mature Stage' of mesocyclone
evolution (just preceding the mesocyclone core's greatest depth). This period of tornadogenesis is earlier compared to
observations recorded by (Burgess et al. 1982) for mesocyclones associated with traditional supercells.
- bowing of the convective line south or southwest of Circ 2 will occur resulting in enhanced wind damage just south of the vortex.
- The 2nd vortex appeared to play a role in transfering momentum from the storm's lower-mid-level region to the surface.
- Circ 2's overall lifespan may vary from 40 to 80 minutes.
- Subseqent 'non-descending' tornadic and non-tornadic vortices often form near or north of the apex of the bowing convective line.
|
(2) Examples of the First and Second Core circulations associated with TYPE 2 events.
|
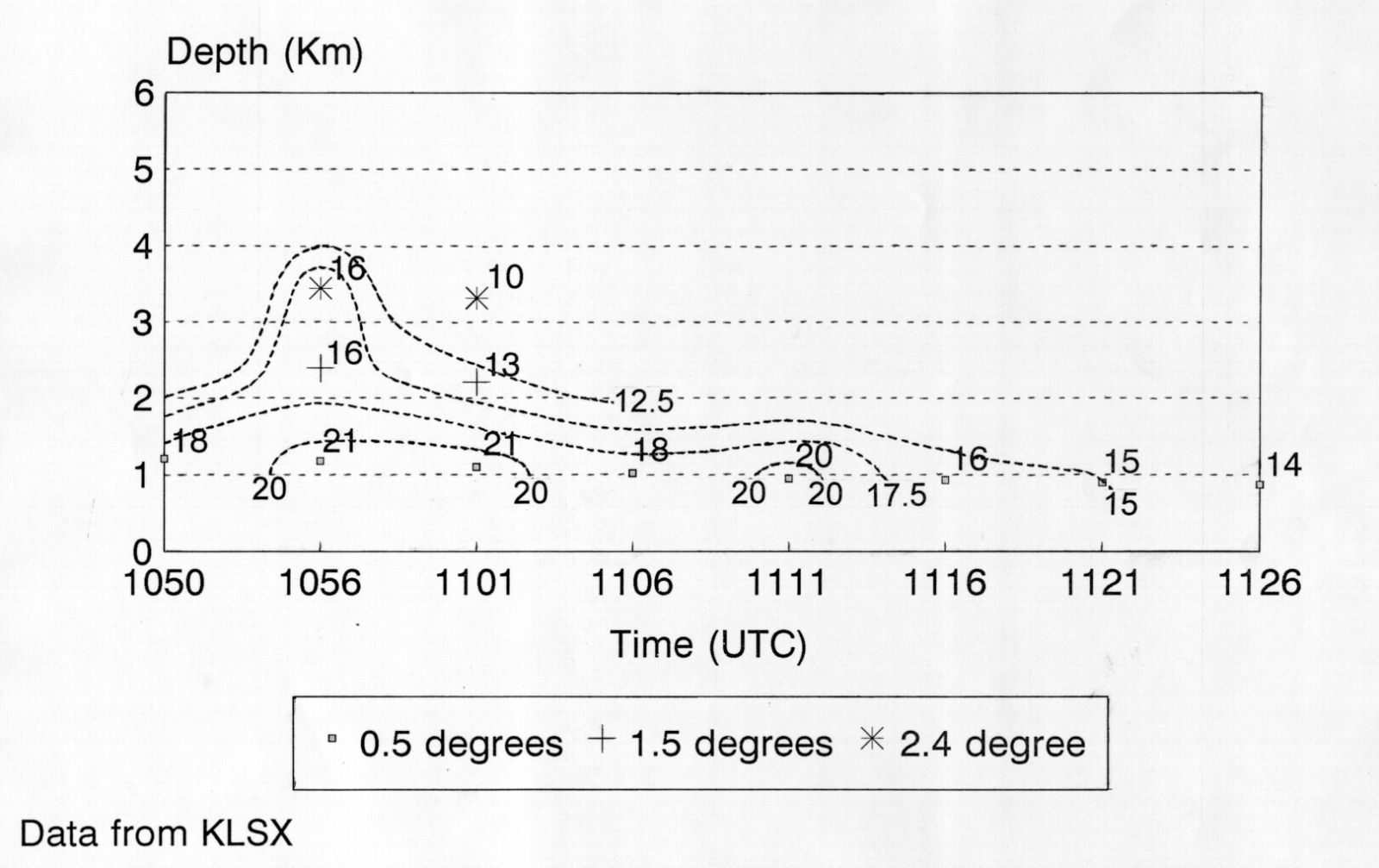
Rotational Velocity (Vr) trace of Circ #1; 14 June 1998.
Vr magnitudes are in m/s.
|
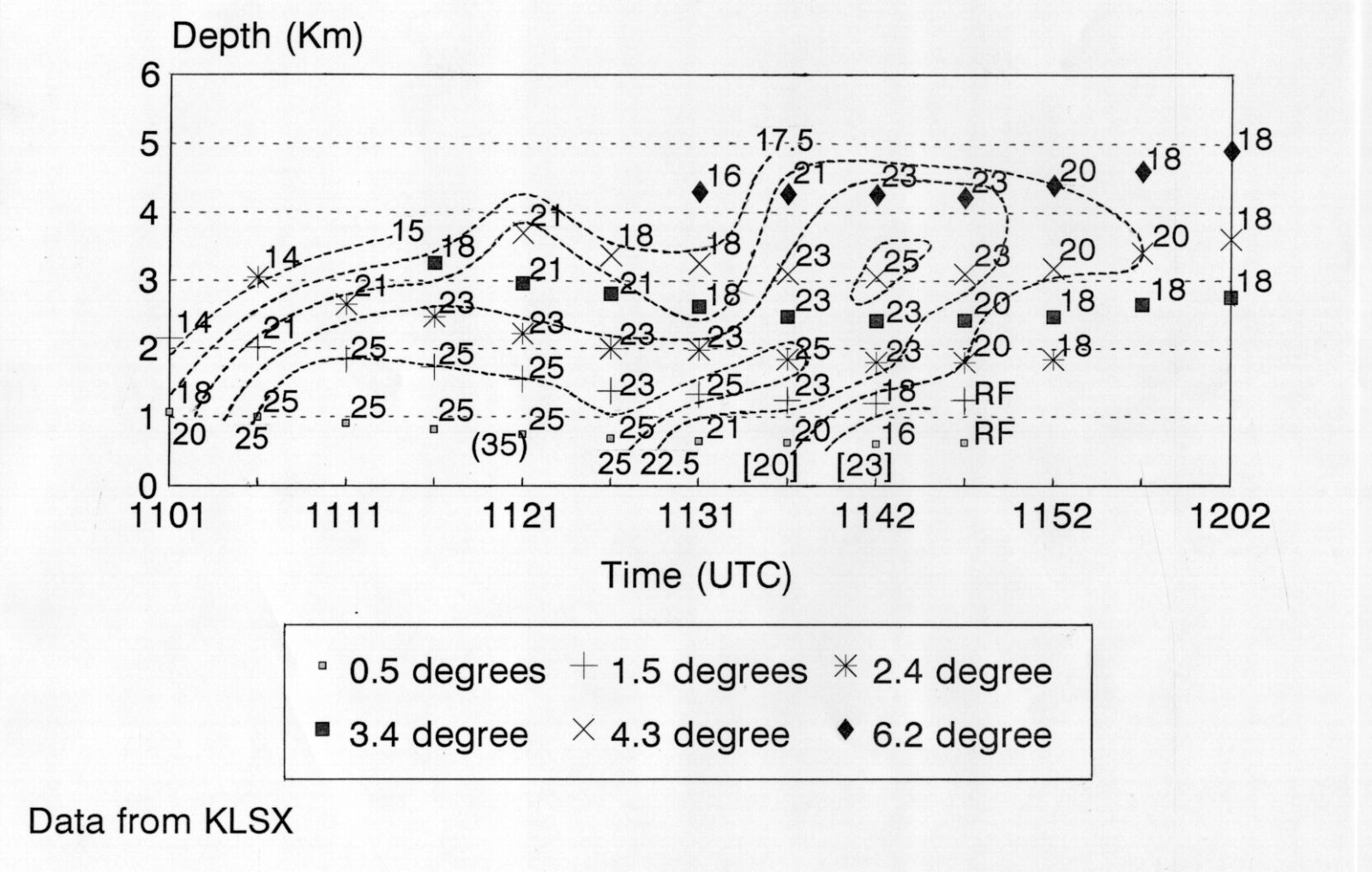
Rotational Velocity (Vr) trace of Circulation #2; 14 June 1998.
Magnitudes of Vr are in m/s.
|
(3) Characteristics of 1st and 2nd core circulations associated with TYPE 2 events (Organizing Stage).
|
TABLE #3a - ORGANIZING STAGE
|
OS (Vr) m/s
Low
|
OS Dia (km) Low
|
OS Vr (km)
|
OS Dia (km)
|
Tornado Occurrence
|
1st CORE
|
17 m/s
|
3.9 km
|
17.5 m/s
|
4.25 km
|
No
|
2nd CORE
|
19 m/s
|
6.0 km
|
18.5 m/s
|
6.1 km
|
Y 1/4-(F0)
|
|
Table #3b - ORGANIZING STAGE: Results from Burgess's Study (Mini/Traditional Supercells) (Burgess et al. 1995). In their study, vortices were sampled up to 150 km for traditional supercells and 98 km for mini-supercells.
Organizing Stage |
OS Vr (m/s) |
OS Dia (km) |
Mini |
13 m/s |
3.9 km |
Traditional |
20 m/s |
5.4 km |
|
(4) Characteristics of 1st and 2nd core circulations associated with TYPE 2 events (Mature Stage).
|
TABLE #4a - MATURE STAGE
|
MS (Vr) m/s
Low |
MS Dia (km) Low |
MS (Vr) m/s |
MS Dia (km) |
Depth (km) |
Tornado Occurrence |
Circulation Lifespan |
1st CORE |
18 m/s |
4.2 - 5.5 km |
17.5 m/s |
4.2 - 6.0 km |
5.6 km |
No |
30 min |
2nd CORE |
21 m/s |
5.8 - 6.6 km |
20.5 m/s |
5.5 - 7.3 km |
7.1 km |
Y 3/4 F0/F1 |
55 min |
|
Table #4b - MATURE STAGE: Results from Burgess's Study (Mini/Traditional Supercells) (Burgess et al. 1998).
Mature Stage |
MS (Vr) m/s Low |
MS Dia (km) Low |
MS (Vr) m/s |
MS Dia (km) |
Depth (km) |
Mini |
15 m/s |
3.5 km |
17 m/s |
3.7 km |
4.5 km |
Traditional |
23 m/s |
5.4 km |
25 m/s |
6.0 km |
9.2 km |
|
Preliminary Findings:
- Similar to the Table #1a, Doppler observations of vortices during the 'Organizing Stage' showed that the 2nd core (core #2) exhibited
stronger Vr shears at both low-levels and overall vortex depth compared to the first core (core #1) (Table 3a).
- Additionally, the 2nd core frequently showed a greater depth and significantly longer lifetime compared to core #1.
- Tornadoes were documented in the vicinity of the 2nd core in 3 of the 4 cases studied. Tornadoes occurred during the later part of
the 'Organizing Stage and very early part of the 'Mature Stage' of mesocylone evolution.
- In comparison to Burgess's results (Tables 3a and 3b), the magnitude of bow echo 2nd core vortices during the 'Organizing Stage'
exhibited overall slightly weaker Vr shears compared to traditional supercell vortices. The overall diameters of the bow echo 2nd
cores were slightly larger compared to core diameters associated with traditional supercell vortices.
- During the mature stage (Tables 4a and 4b), the magnitude of bow echo 2nd core vortices (low-levels) exhibited slightly weaker
magnitudes of Vr shears compared to traditional supercell vortices. The low-level diameters were slightly larger compared to
traditional supercell core diameters. When comparing the Vr magnitudes of the overall vortex depth, the bow echo 2nd core vortices
were weaker (20.5 m/s) compared to (25 m/s) for traditional supercell vortices. In contrast, the core diameters of both 2nd core
vortices and traditional supercell vortices were relatively similar.
In comparing the overall vortex depth between the two groups, bow echo 2nd core vortices exhibited a slightly smaller depth (7.1 km)
to traditional supercell vortices (9.2 km).
- In most of our Type 1 and Type 2 cases, the 'external boundary appeared to serve as a source of local vorticity to aid in
mesocyclone intensification. This vorticity is often tapped by the storm's updraft resulting in vertical stretching within that part of the
convective updraft structure. End result is genesis and rapid intensification of (non-tornadic or tornadic) vortices in the vicinity of the
intersection between the external boundary and convective line.
- Subsequent (tornadic or non-tornadic) vortices which form near or north of the apex of the bowing structure appeared to result from
shearing instabilities/strong horizontal shears along the leading edge of an advancing gust front/bowing convective line.
BACK TO MCS Intro
|